Various sugars impact yeast respiration differently in experiments. Glucose, a simple sugar, is readily fermented by yeast, leading to efficient respiration. Fructose, another simple sugar, also supports yeast activity but at a slightly slower rate compared to glucose. Sucrose, a disaccharide of glucose and fructose, requires an extra enzymatic step for yeast to break down, causing delayed but sustained respiration.
Complex sugars like maltose may undergo slower fermentation due to the need for additional enzymatic processes. Lactose, found in dairy, is less favorable for yeast respiration, as yeast typically lacks the required enzymes to break it down efficiently. This blog article will continue with a discussion on yeast respiration relative to different kinds of sugars.
Glucose Respiration Efficiency:
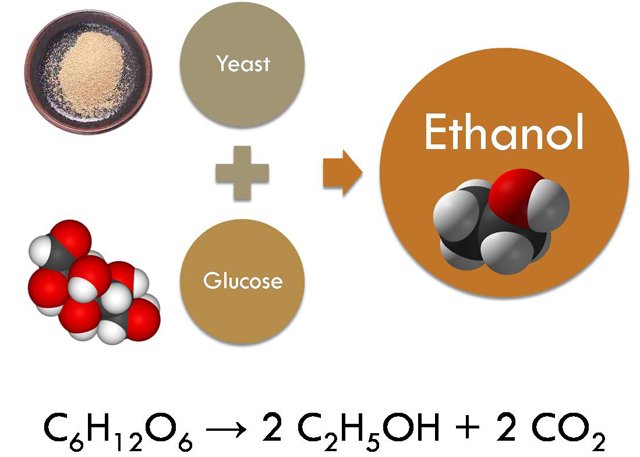
In yeast respiration, glucose emerges as a pivotal player, driving efficiency in the fermentation process. As a simple sugar, glucose is readily metabolized by yeast, initiating a cascade of biochemical reactions that swiftly propel yeast respiration. The key lies in the straightforward structure of glucose, which facilitates its rapid uptake and conversion within yeast cells.
Upon introduction, glucose acts as a prime substrate for yeast, instigating a rapid and efficient respiration process. The simplicity of its molecular composition allows yeast cells to swiftly break down glucose into smaller, energy-yielding molecules, such as ATP. This expeditious energy production fuels the cellular processes driving yeast respiration, contributing to a pronounced and prompt impact.
Fructose’s Role in Yeast Activity:
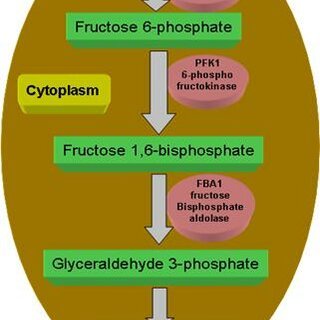
Turning our attention to another simple sugar, fructose reveals a nuanced dynamic in yeast respiration. While less swiftly metabolized than glucose, fructose still plays a significant role in supporting yeast activity. Though similar to glucose, the molecular structure of fructose introduces subtle variations that impact the rate at which yeast cells can harness its energy.
As yeast encounters fructose, the slightly slower pace of fermentation becomes evident. Fructose undergoes a series of enzymatic transformations within yeast cells, leading to the release of energy in the form of ATP. Although the rate is not as rapid as with glucose, fructose’s contribution to sustaining yeast respiration showcases the adaptability of these microorganisms to different sugar substrates.
By discerning the distinctive characteristics of fructose metabolism, researchers gain insights into the diverse strategies yeast employs to extract energy from various simple sugars.
Sucrose and Enzymatic Steps:
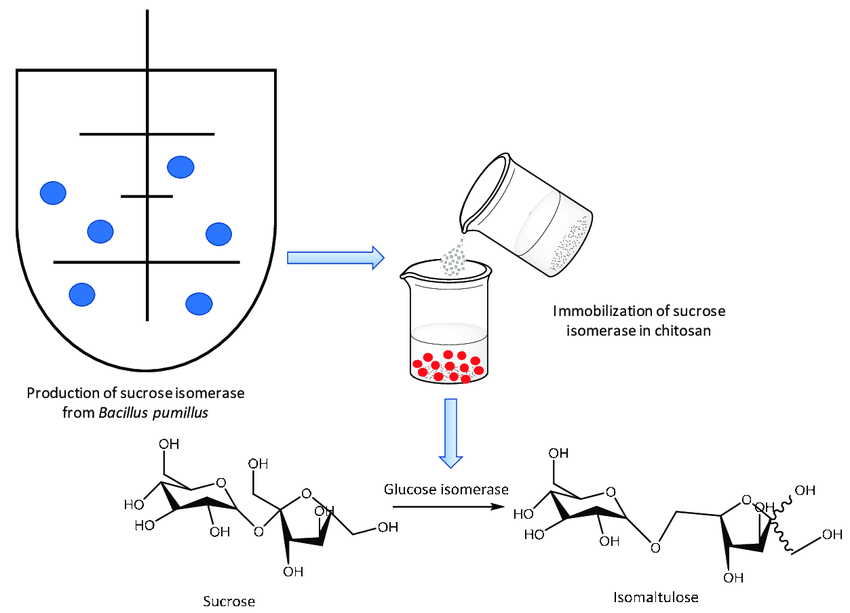
Sucrose, a disaccharide composed of glucose and fructose, introduces an additional layer of complexity to yeast respiration. Unlike simple sugars, sucrose necessitates an extra enzymatic step for yeast cells to break it down into its constituent glucose and fructose molecules. This enzymatic process, sucrose cleavage, adds a temporal dimension to the respiration timeline.
Upon the introduction of sucrose, yeast cells initiate the production of the enzyme invertase. This enzyme facilitates the cleavage of sucrose into its simpler components, glucose, and fructose. The sequential enzymatic steps in sucrose breakdown contribute to delayed yet sustained yeast respiration. The time required for the enzymatic processes underscores the intricate nature of disaccharide metabolism within yeast cells.
Examining sucrose and its enzymatic steps expands our understanding of how yeast adapts to diverse sugar substrates. The additional complexity introduced by disaccharides like sucrose highlights the importance of considering the specific metabolic pathways involved in yeast respiration when dealing with more intricate sugar structures.
Challenges of Maltose Fermentation:
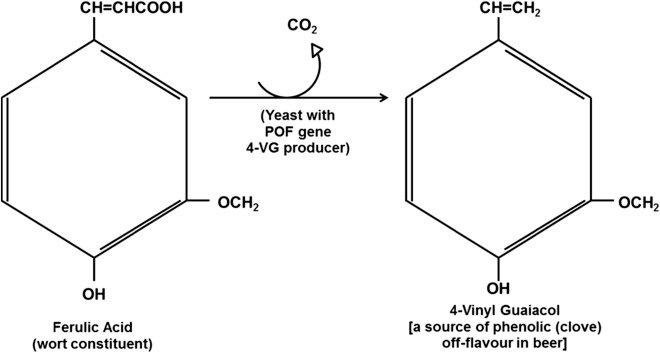
Maltose, a complex sugar of two glucose molecules, presents distinct challenges in yeast respiration. Unlike simpler sugars, maltose undergoes a slower fermentation process due to the need for additional enzymatic steps. The presence of maltase, an enzyme involved explicitly in breaking down maltose, becomes crucial for yeast cells to extract energy efficiently.
When maltose is introduced, yeast cells must first produce maltase to catalyze its breakdown into individual glucose molecules. This enzymatic step delays the overall fermentation process, making maltose a less readily available energy source than simpler sugars. The slower fermentation of maltose sheds light on the intricacies of yeast metabolism and its ability to adapt to varying sugar complexities.
By unraveling the specific hurdles posed by complex sugars like maltose, researchers gain insights into the regulatory mechanisms and enzymatic adaptations that govern yeast respiration in diverse nutritional environments.
Lactose’s Impact on Yeast:
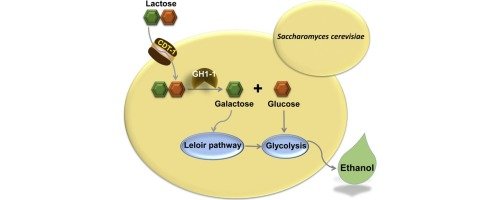
Incorporating lactose, a sugar commonly found in dairy products, into the yeast respiration experiment reveals distinctive challenges. Unlike glucose or fructose, yeast cells typically lack the requisite enzymes, such as lactase, to break down lactose efficiently. This deficiency leads to a less favorable environment for yeast respiration when lactose is the primary sugar source.
Upon the introduction of lactose, yeast cells may struggle to hydrolyze it into its constituent sugars, glucose, and galactose. The limited enzymatic machinery for lactose metabolism results in a comparatively slower and less efficient fermentation process. This aspect highlights the specificity of yeast cells in utilizing sugars, with lactose presenting a less preferred substrate for energy production.
Analyzing lactose’s impact on yeast contributes to a comprehensive understanding of the limitations and preferences within yeast metabolism. Recognizing the challenges of sugars like lactose underscores the importance of tailored experimental conditions when investigating yeast respiration in diverse nutritional contexts.
Comparative Analysis of Sugar Types:
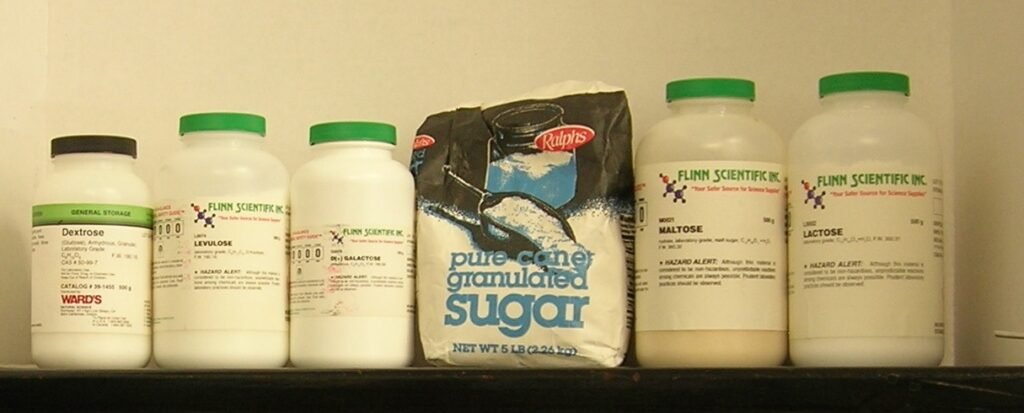
A comparative analysis of the various sugars in the experiment broadcasts intriguing insights into yeast respiration dynamics. By juxtaposing the performance of glucose, fructose, sucrose, maltose, and lactose, a vivid understanding of the effect of different sugars on yeast respiration experiment emerges.
Speed of Respiration: Investigate and compare the rates at which yeast cells respire when exposed to different sugars, highlighting the variations in the speed of energy extraction.
Enzymatic Efficiency: Assess the efficiency of enzymatic processes involved in breaking down each sugar, emphasizing the adaptability of yeast cells to different enzymatic requirements.
Sustainability of Respiration: Examine the sustainability of yeast respiration over time with each sugar, shedding light on the duration and consistency of energy production.
Quantitative Energy Yield: Quantify the energy yield in terms of ATP production for each sugar, providing a quantitative measure of the metabolic efficiency of yeast in utilizing different substrates.
Cellular Adaptations: Explore any observed cellular adaptations or changes in yeast morphology in response to different sugars, indicating the dynamic nature of yeast metabolism.
Insights into Metabolic Processes:
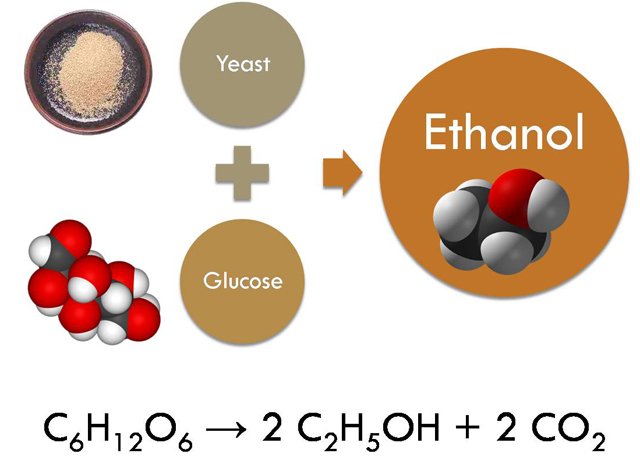
As we conclude our discussion, we must draw overarching insights into the metabolic processes governing yeast respiration in response to different sugars. The following are key findings from the experiment that provide a holistic view of the metabolic adaptations and nuances observed within yeast cells.
Metabolic Flexibility: Highlight how yeast showcases metabolic flexibility by efficiently adapting to diverse sugar substrates, demonstrating its ability to modulate respiration strategies based on nutritional availability.
Enzymatic Plasticity: Emphasize the plasticity of yeast enzymes, showcasing their capacity to adjust to the specific enzymatic requirements of different sugars, ranging from simple to complex structures.
Energy Optimization: Discuss how yeast appears to optimize energy production based on the intrinsic properties of each sugar and balancing factors such as speed, sustainability, and overall ATP yield.
Nutritional Preferences: Address any observed preferences yeast may exhibit for certain sugars, shedding light on the dietary choices that optimize cellular function.
Implications for Biotechnology: Consider the broader implications of the findings for biotechnological applications, such as industrial fermentation processes that leverage yeast for various purposes.
Conclusion:
In conclusion, the effect of different sugars on yeast respiration experiments varies according to the inherent characteristics of each sugar. Molecular structure, simplicity, and enzymatic processes influence yeast respiration’s speed, efficiency, and sustainability. Simple sugars like glucose exhibit rapid and efficient fermentation, while the complexity of disaccharides and lactose presents challenges, leading to slower and less favorable outcomes. This nuanced understanding provides a foundation for tailoring experimental conditions and offers valuable insights into the convoluted interplay between sugar types and yeast metabolic responses.